In a new study, researchers have taken an important step toward understanding how exploding stars can help reveal how neutrinos, mysterious subatomic particles, secretly interact with themselves.
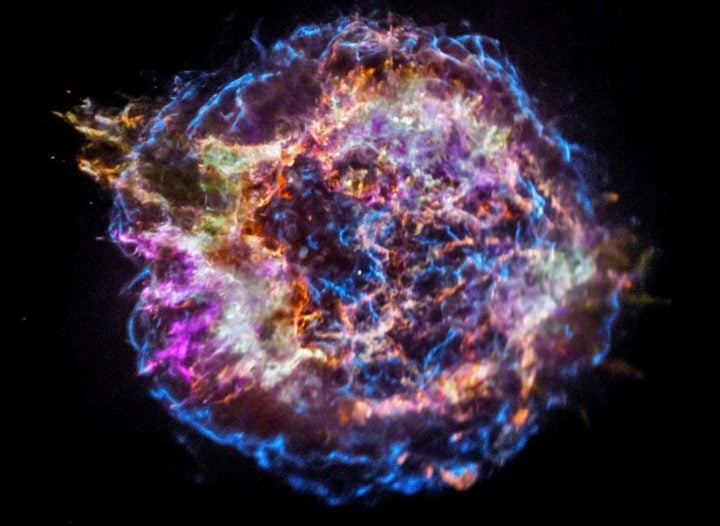
Cassiopeia A (Cas A), a supernova remnant – illustrative image. These celestial objects are the sources of neutrinos. Credits: NASA/CXC/SAO
One of the less well-understood elementary particles, neutrinos rarely interact with normal matter, and instead travel invisibly through it at almost the speed of light.
These ghostly particles outnumber all the atoms in the universe and are always passing harmlessly through our bodies, but due to their low mass and lack of an electric charge they can be incredibly difficult to find and study.
But in a study published in the journal Physical Review Letters, researchers at The Ohio State University have established a new framework detailing how supernovae – massive explosions that herald the death of collapsing stars – could be used as powerful tools to study how neutrino self-interactions can cause vast cosmological changes in the universe.
“Neutrinos only have very small rates of interaction with typical matter, so it’s difficult to detect them and test any of their properties,” said Po-Wen Chang, the study’s lead author and a physics graduate student at Ohio State.
“That’s why we have to use astrophysics and cosmology to discover interesting phenomena about them.”
Thought to have been important to the formation of the early universe, neutrinos are still puzzling to scientists, despite having learned that they originate from a number of sources, such as in nuclear reactors or the insides of dying stars.
But by calculating how self-interactions would affect the neutrino signal from Supernova 1987A, the nearest supernova observed in modern times, researchers found that when neutrinos do interact with themselves, they form a tightly coupled fluid that expands under relativistic hydrodynamics – a branch of physics that deals with how flows impact solid objects in one of two different ways.
In the case of what’s called a “burst outflow,” the team theorizes that much like popping a highly pressurized balloon in the vacuum of space would push energy outward, a burst produces a neutrino fluid that moves in all directions.
The second case, described as a “wind outflow,” imagines a highly pressurized balloon with many nozzles, wherein neutrinos escape at a more constant flow rate, similar to a jet of steady wind.
While the wind-outflow theory is more likely to take place in nature, said Chang, if the burst case is realized, scientists could see new observable neutrino signatures emitted from supernovae, allowing unprecedented sensitivity to neutrino self-interactions.
One of the reasons it’s so vital to understand these mechanisms is that if neutrinos are acting as a fluid, that means they are acting together, as a collective.
And if the properties of neutrinos are different as a collective than individually, then the physics of supernovae could experience changes too. But whether these changes are due solely to the burst case or the outflow case remains to be seen.
“The dynamics of supernovae are complicated, but this result is promising because with relativistic hydrodynamics we know there’s a fork in the road in understanding how they work now,” said Chang.
Still, further research needs to be done before scientists can cross off the possibility of the burst case happening inside supernovae as well.
Despite these uncertainties, the study is a huge milestone in answering the decades-old astrophysical issue of how neutrinos actually scatter when ejected from supernovae, said John Beacom, co-author of the study and a professor of physics and astronomy at Ohio State.
This study found that in the burst case, unprecedented sensitivity to neutrino self-interactions is possible even with sparse neutrino data from SN 1987A and conservative analysis assumptions.
“This problem has lain basically untouched for 35 years,” said Beacom. “So even though we were not able to completely solve how neutrinos affect supernovae, what we’re excited about is that we were able to make a substantial step forward.”
Down the road, the team hopes their work will be used as a stepping stone to further investigate neutrino self-interactions. Yet because only about two or three supernovae happen per century in the Milky Way, it’s likely researchers will have to wait decades more to collect enough new neutrino data to prove their ideas.
“We’re always praying for another galactic supernova to happen somewhere and soon, but the best we can do is try to build on what we know as much as possible before it happens,” said Chang.
Source: Ohio State University