A team at HZB has developed a new measurement method that, for the first time, accurately detects tiny temperature differences in the range of 100 microKelvin in the thermal Hall effect. Previously, these temperature differences could not be measured quantitatively due to thermal noise.
Their study is published in Materials & Design.
Using the well-known terbium titanate as an example, the team demonstrated that the method delivers highly reliable results. The thermal Hall effect provides information about coherent multi-particle states in quantum materials based on their interaction with lattice vibrations (phonons).
The laws of quantum physics apply to all materials. However, these laws give rise to particularly unusual properties in so-called quantum materials. For example, magnetic fields or changes in temperature can cause excitations, collective states, or quasiparticles that are accompanied by phase transitions to exotic states.
This can be utilized in a variety of ways, provided it can be understood, managed, and controlled. For example, in the future, information technologies that can store or process data with minimal energy requirements.
The thermal Hall effect (THE) plays a key role in identifying exotic states in condensed matter. The effect is based on tiny transverse temperature differences that occur when a thermal current is passed through a sample and a perpendicular magnetic field is applied.
In particular, the quantitative measurement of the thermal Hall effect allows us to separate the exotic excitations from conventional behavior. The thermal Hall effect is observed in a variety of materials, including spin liquids, spin ice, parent phases of high-temperature superconductors, and materials with strongly polar properties.
However, the thermal differences that occur perpendicular to the temperature gradient in the sample are extremely small: in typical millimeter-sized samples, they are in the range of microkelvins to millikelvins. Until now, it has been difficult to detect these heat differences experimentally because the heat introduced by the measurement electronics and sensors masks the effect.
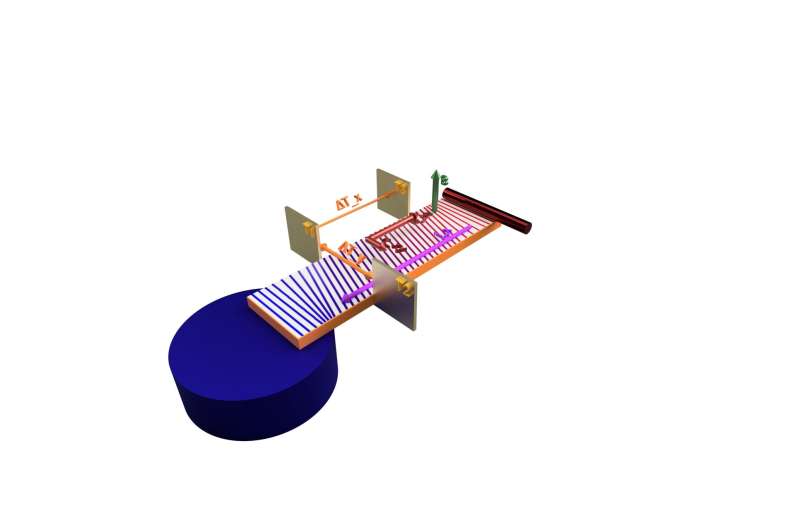
A novel sample holder
The team led by PD Dr. Klaus Habicht has now carried out pioneering work. Together with specialists from the HZB sample environment, they have developed a novel sample rod with a modular structure that can be inserted into various cryomagnets. The sample head measures the thermal Hall effect using capacitive thermometry.
This takes advantage of the temperature dependence of the capacitance of specially manufactured miniature capacitors. With this setup, the experts have succeeded in significantly reducing heat transfer through sensors and electronics and in attenuating interference signals and noise with several innovations.
To validate the measurement method, they analyzed a sample of terbium titanate, whose thermal conductivity in different crystal directions under a magnetic field is well known. The measured data were in excellent agreement with the literature.
Further improvement of the measurement method
“The ability to resolve temperature differences in the sub-millikelvin range fascinates me greatly and is a key to studying quantum materials in more detail,” says first author Dr. Danny Kojda. “We have now jointly developed a sophisticated experimental design, clear measurement protocols, and precise analysis procedures that allow high-resolution and reproducible measurements.”
Department head Klaus Habicht adds, “Our work also provides information how to further improve the resolution in future instruments designed for low sample temperatures. I would like to thank everyone involved, especially the sample environment team. I hope that the experimental setup will be firmly integrated into the HZB infrastructure and that the proposed upgrades will be implemented.”
Habicht’s group will now use measurements of the thermal Hall effect to investigate the topological properties of lattice vibrations or phonons in quantum materials.
“The microscopic mechanisms and the physics of the scattering processes for the thermal Hall effect in ionic crystals are far from being fully understood. The exciting question is why electrically neutral quasiparticles in non-magnetic insulators are nevertheless deflected in the magnetic field,” says Habicht. With the new instrument, the team has now created the prerequisites to answer this question.
More information:
Danny Kojda et al, Advancing the precision of thermal Hall measurements for novel materials research, Materials & Design (2023). DOI: 10.1016/j.matdes.2023.112595
Citation:
Higher measurement accuracy opens new window to the quantum world (2024, January 17)
retrieved 17 January 2024
from
This document is subject to copyright. Apart from any fair dealing for the purpose of private study or research, no
part may be reproduced without the written permission. The content is provided for information purposes only.