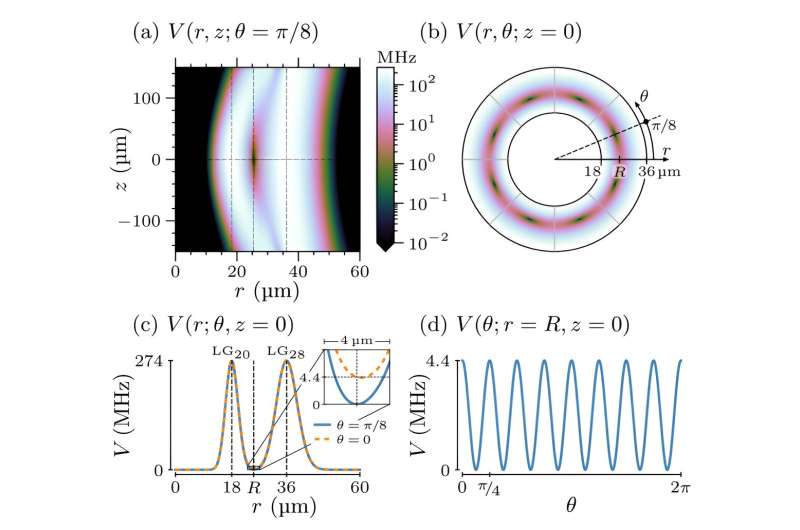
from LG20 and LG28 laser modes with λ = 795 nm and respective Gaussian waists of w0,1 = 5.78 µm and w0,2 = 9.66 µm,
and powers of P1 = 5.38 mW and P2 = 17.78 mW. The
beams are assumed to be blue-detuned for an AC polarizability of −0.16 Hz/(V/m)2
. (a) Optical potential in the r−z
plane. (b) Optical potential in the transverse plane at the
focus z = 0. (c) Potential along the radial direction for z = 0
and θ = 0 and π/8. (d) Potential along the azimuthal direction at R = 25.46 µm and z = 0.Credit: arXiv: DOI: 10.48550/arxiv.2309.06324
Atom interferometers are quantum sensors that use the wave-like nature of atoms to measure gravity, acceleration and rotation with exceptional precision.
Most of the current atom interferometers are large instruments, occupying buildings and requiring towers that can reach tens of meters in height. Now, University of Michigan physicists have developed a design for a quantum rotation sensor with a core size that is barely visible to the human eye.
The proof-of-concept design could help bring atom interferometer-based quantum sensors out of the laboratory and into the world, according to lead author and U-M doctoral student Bineet Dash.
Scientists could use atom interferometers in quests ranging from the continual hunt for the tiny ripples in the fabric of our universe caused by gravitational waves to understanding minute, localized changes in Earth’s gravity caused by melting ice sheets in Antarctica, Dash says. But because of their size, atom interferometers are typically bound to laboratory settings. Currently, the most sensitive atom interferometers use tall towers inside buildings to shoot beams of atoms across tens of meters to gather information.
The new design developed by Dash and the lab of U-M physicist Georg Raithel uses a special kind of laser beam that traps atoms in a pinwheel-shaped geometry, which can be scaled from a 30 micron radius, smaller than the diameter of a human hair, and up to about 10 times larger, about 300 microns. The researchers’ design is published in the journal AVS Quantum Science.
“This interferometer is not an incremental improvement on existing designs that were developed elsewhere,” Dash said. “This is based on a fundamentally different approach proposed by our group in 2021.”
Currently, researchers often use interferometers that are based on laser-wave interference. In astrophysics, such optical interferometers are employed to detect gravitational waves, Dash said. In inertial navigation, optical interferometers are used to measure the rotation of airplanes, ships and satellites.
“People often say that there is already a sensor that works with light. Why do we need to develop a sensor that uses quantum mechanics?” Dash said. “A major motivation is that atom interferometers can be orders of magnitude more sensitive than optical interferometers under the same conditions.”
Light-interferometer-based rotation sensors use what’s called the Sagnac effect. French physicist Georges Sagnac discovered that light could be used to measure rotation: If you send light around a rotating body, then send another stream of light counter to the rotation, the waves of light overlap. But these waves of light “interfere” with each other and begin to show a difference in the path that they travel. This difference can be used to measure the speed of rotation.
Atom-interferometric rotation sensors are based on the same concept. According to quantum mechanics, atoms are particles, but they also behave like waves. Because their wavelength is much smaller than that of light, according to Dash, they allow for more accurate and sensitive rotation measurement than light interferometers.
But in addition to their large size, the way a majority of existing atom interferometers operate also presents problems for their use outside of the lab. Atoms are shot up into a vacuum within a laboratory tower on separate paths using laser pulses, Dash said. One atomic path reaches higher than the other, and then they meet back at the bottom. The lag between when they meet gives information about the background acceleration.
When atoms are set loose into free space, they can disperse, and when they disperse, information is lost. At some point, atoms will disperse enough that all information is lost. And although the atom streams are launched together, many atoms don’t meet back, which leads to more missed information.
In 2021, Raithel’s lab, in which Dash is a student, used the idea of “optical lattices” to try to scale down the size of atom interferometers. Optical lattices are created when two laser beams moving in opposite directions cross paths giving rise to grids of light. Under appropriate conditions, atoms can be trapped at the minima of the light grid, like eggs sitting in an egg carton, Dash said. The optical lattices keep the atoms confined and allow experimenters to guide the atoms on predetermined paths that are certain to meet back again.
The 2021 study showed that atoms in different quantum states can be trapped in separate lattices by carefully tuning the laser parameters. Using laser pulses, the researchers can craft a quantum superposition of states trapped in two such optical lattices. Moving these two lattices in opposite directions creates a lag between the two streams of atoms, which can then be used to read background acceleration.
But the 2021 design only sent atoms back and forth along a straight line, which is not amenable for rotation sensing.
In the current study, Raithel’s lab devised a way to use a special kind of laser beam that sends atoms in an angular pattern rather than a linear standing wave pattern.
“It looks like a pinwheel, and by changing the laser frequencies slightly, the speed of the pinwheel can be changed,” Dash said. “After a predetermined time of spinning, a lag develops between the pinwheels, and we can use this lag to figure out the background rotation.”
Dash said that while the paper describes a proof-of-concept design, the Raithel lab is currently working on a prototype experiment that utilizes a source of Bose condensed cold atoms.
“Current atom interferometers are great for fundamental physics, but they are heavy, power intensive, take up a lot of space, and essentially because of their geometric footprint, they are not practically useful,” Dash said.
“Our study is about prototype development. But it is a very general technique that can be adapted for practical applications in, for instance, inertial navigation and gravity mapping, as well as for research in fundamental physics.”
More information:
Bineet Dash et al, Rotation sensing using tractor atom interferometry, AVS Quantum Science (2024). DOI: 10.1116/5.0175802. On arXiv: DOI: 10.48550/arxiv.2309.06324
Citation:
Proof-of-concept design shrinks quantum rotation sensor to micron scale (2024, October 1)
retrieved 1 October 2024
from
This document is subject to copyright. Apart from any fair dealing for the purpose of private study or research, no
part may be reproduced without the written permission. The content is provided for information purposes only.