Quantum information (QI) processing may be the next game changer in the evolution of technology, by providing unprecedented computational capabilities, security and detection sensitivities. Qubits, the basic hardware element for quantum information, are the building block for quantum computers and quantum information processing, but there is still much debate on which types of qubits are actually the best.
Research and development in this field is growing at astonishing paces to see which system or platform outruns the other. To mention a few, platforms as diverse as superconducting Josephson junctions, trapped ions, topological qubits, ultra-cold neutral atoms, or even diamond vacancies constitute the zoo of possibilities to make qubits.
So far, only a handful of qubit platforms have been demonstrated to have the potential for quantum computing, marking the checklist of high-fidelity controlled gates, easy qubit-qubit coupling, and good isolation from the environment, which means sufficiently long-lived coherence.
Nano-mechanical resonators may be a part of the handful of platforms. They are oscillators, like springs and strings (e.g. guitars) that when driven, create harmonic or anharmonic sounds depending on the strength of the drive. But what happens when we cool a nano resonator down to absolute zero temperature?
The energy levels of the oscillator becomes quantized and the resonator vibrates with its characteristic zero point motion. The zero-point motion arises from the Heisenberg uncertainty principle. In other words, a resonator maintains motion even when it is in the ground state. The realization of a mechanical qubit is possible if the quantized energy levels of a resonator are not evenly spaced.
The challenge is to keep the nonlinear effects big enough in the quantum regime, where the oscillator’s zero point displacement is miniscule. If this is achieved, then the system may be used as qubit by manipulating it between the two lowest quantum levels without driving it in higher energy states.
For many years, there has been a lot of interest in realizing a qubit system with a mechanical nano resonator. In 2021, Fabio Pistolesi (Univ. Bordeaux-CNRS), Andrew N. Cleland (Univ. Chicago), and ICFO Prof. Adrian Bachtold, established a solid theoretical concept of a mechanical qubit, based on a nanotube resonator coupled to a double-quantum dot under an ultrastrong coupling regime.
These theoretical results proved that these nanomechanical resonators could indeed become ideal candidates for qubits. Why? Because they have been shown to feature long coherence times, a definite “must” for quantum computing.
Taking into account that there was a theoretical framework to work with, the challenge now was to actually make a qubit out of a mechanical resonator, and find the appropriate conditions and parameters to control the non-linearities in the system.
After several years of endless work on these systems, the challenges of experimentally realizing this has given its first very welcomed green light. In a recent study published in Nature Physics, ICFO researchers Chandan Samanta, Sergio Lucio de Bonis, Christoffer Moller, Roger Tormo-Queralt, W. Yang, Carles Urgell, led by ICFO Prof. Adrian Bachtold, in collaboration with researchers B. Stamenic and B.Thibeault from University of California Santa Barbara, Y. Jin from Université Paris-Saclay-CNRS, D.A. Czaplewski from Argonne National Laboratory, and F. Pistolesi from Univ. Bordeaux-CNRS, achieved the first pre-experimental steps for the future realization of a mechanical qubit by demonstrating a new mechanism to boost the anharmonicity of a mechanical oscillator in its quantum regime.
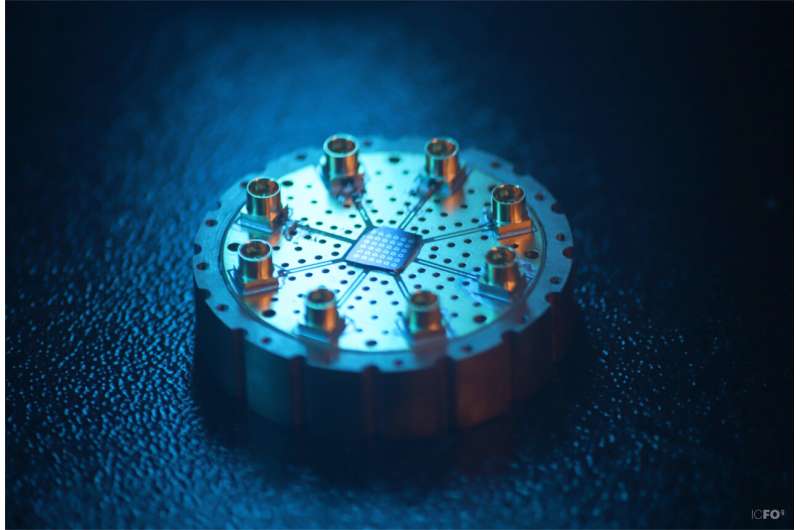
The experiment: Engineering anharmonicity close to the ground state
The team of researchers fabricated a suspended nanotube device of approximately 1.4 micrometers in length, with its extremes hooked onto the edges of two electrodes. They defined a quantum dot which is a two level electronic system on the vibrating nanotube by electrostatically creating tunnel junctions at both ends of the suspended nanotube.
Then, by adjusting the voltage on the gate electrode, they allowed the flow of only one electron at a time onto the nanotube. The mechanical motion of the nanotube was then coupled to the single electron in the single electron tunneling regime. This electromechanical coupling created anharmonicity to the mechanical system.
Subsequently, they decreased the temperature down to mK (milikelvins, almost absolute zero) and entered into an ultra-strong coupling regime where each additional electron on the nanotube shifted the equilibrium position of the nanotube away from its zero-point amplitude. With an amplitude only a factor of 13 over the zero-point motion, they were able to notice these nonlinear vibrations.
The results are astonishing because vibrations present in other resonators, cooled to the quantum ground state, showed to only be nonlinear at amplitudes approximately 106 times greater than its zero-point motion.
This new mechanism displays remarkable physics because, contrary to what was expected, the anharmonicity increases as the vibrations are cooled closer to the ground state. This is just the opposite of what has been observed in all other mechanical resonators so far. As first author Chandan Samanta says, “when researchers first began studying nanomechanical resonators, a recurrent question was whether it would be possible to achieve nonlinearities in vibrations that are in the quantum ground state.”
“Some leading researchers in the field argued that this would be a challenging feat due to technological limitations, and this view has remained the accepted paradigm until now. In this context, our work represents a significant conceptual advance because we demonstrate that nonlinear vibrations in the quantum regime are indeed achievable.”
“We are confident that the nonlinear effects could have been further enhanced by getting closer to the quantum ground state, but we were limited by the temperature of our current cryostat. Our work provides a roadmap for achieving nonlinear vibrations in the quantum regime.”
Contrary to what has been observed so far in other mechanical resonators, the team of researchers found a method to boost the anharmonicity of a mechanical oscillator near its quantum regime. The results of this study set the first stepping stones for the future development of mechanical qubits or even quantum simulators.
As Adrian Bachtold remarks, “It is remarkable that we entered into ultra-strong coupling regime and observed strong anharmonicity in the resonator. But the damping rate becomes large at low temperature due to the coupling of the resonator to one quantum dot.”
“In future experiments that target cat states and mechanical qubits, it will be advantageous to couple nanotube vibrations to a double-quantum dot, since it enables strong nonlinearities together with long-lived mechanical states. The damping arising from the electron in the double-quantum dot is exponentially suppressed at low temperature, so that it should be possible to achieve damping rate of 10 Hz measured in nanotubes at low temperature.”
More information:
C. Samanta et al, Nonlinear nanomechanical resonators approaching the quantum ground state, Nature Physics (2023). DOI: 10.1038/s41567-023-02065-9
Citation:
Research takes first steps towards realizing mechanical qubits (2023, June 9)
retrieved 10 June 2023
from
This document is subject to copyright. Apart from any fair dealing for the purpose of private study or research, no
part may be reproduced without the written permission. The content is provided for information purposes only.