Laser-cooled atomic gases, gases of atoms chilled to temperatures around absolute zero using laser technologies, have proved to be versatile physical platforms to study and control quantum phenomena. When these atomic gases interact with light inside an optical cavity (i.e., a structure designed to trap and enhance light), they can give rise to effects that can be leveraged to realize quantum sensing or simulate complex quantum systems.
Using atomic gases loaded in optical cavities, physicists have observed various intriguing effects, including self-organization phase transitions, characterized by the spontaneous arrangement of the gas atoms into ordered patterns, lasing and the preservation of quantum coherence. Generally, however, these effects are only observed for short times, as new atoms need to be reloaded in the cavity for them to be produced again.
Researchers at JILA, a joint research institute of the University of Colorado-Boulder and the National Institute of Standards and Technology, recently demonstrated continuous lasing that lasted hours using laser-cooled strontium-88 (88Sr) atoms loaded into a ring (i.e., circular) optical cavity. Their paper, published in Nature Physics, could open new possibilities for the development of ultra-quiet lasers, as well as quantum computers and sensing technologies.
“The original goal of our experiment is to build a continuous superradiant laser, a tool that would allow us to make high precision frequency measurements at short timescales,” Dr. Vera M. Schäfer, first author of the paper, told Phys.org. “This could help us to explore different regimes to search for dark matter and other new physics.”
The long-term objective of researchers Schäfer, Niu, Thompson and their colleagues is to realize exceedingly advanced and ultranarrow frequency linewidth lasers, which could be used to search for dark matter or to develop sophisticated devices, such as atomic clocks. While working towards this goal, however, they uncovered a curious and unexpected effect, which reflects the fact that nature can spontaneously organize itself when energy is pumped into a system.
“We saw laser light coming out of our system when we were just trying to load a very cold gas of atoms between the highly reflective mirrors that form our laser cavity,” explained Prof. James K. Thompson. “To be clear, our laser cavity is like a bell but for light instead of sound. It likes to ring at a specific frequency.
“The atoms were making this ‘bell’ ring and give off light. When we investigated where this light was coming from, we found lots of strange behaviors, chief among them that changing the resonance frequency of the bell barely changed the frequency of the light it was emitting.”
After their unexpected observations, the researchers set out to better understand the underlying physics. This could, in turn, inform the future development of atomic clocks and gravitational wave detectors.
“To understand this, I have to tell you a story about atomic clocks and gravitational wave detectors,” said Thompson. “It turns out that both atomic clock and gravitational wave detectors rely on building types of optical cavities with very, very, very (did I say very enough?) stable frequencies.
“Yet when one builds these objects, one notices that it looks like these ‘bells’ are wiggling and jiggling around in frequency. Why? Because they are made of real atoms at finite temperatures that undergo the equivalent of random jiggling around called Brownian motion.”
To circumvent this limitation of both atomic clocks and gravitational wave detectors, Thompson and his lab at JILA are trying to build a superradiant laser. The frequency of this laser should not depend on the frequency of the optical cavity but, instead, on a very narrow frequency atomic transition in the atom strontium.
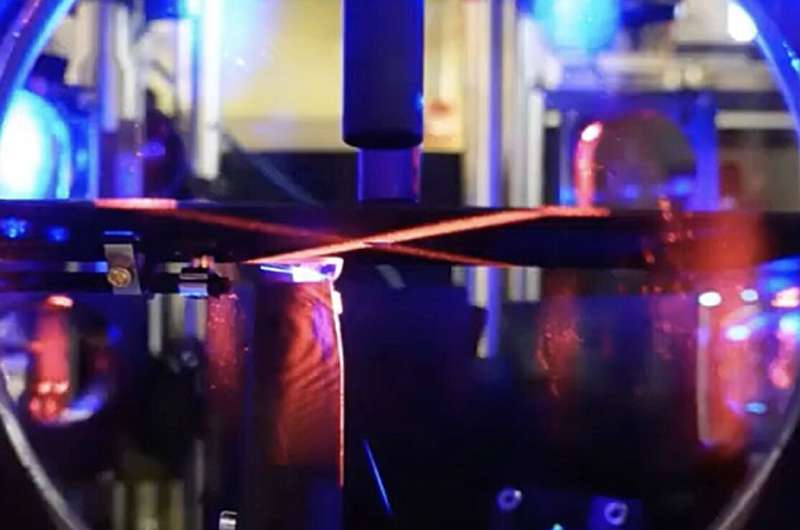
“To build this, we must continuously apply other normal lasers that cool the strontium atoms down to 10 millionths of a degree above absolute zero,” said Thompson.
Senior PhD student Zhijing Niu added, “We have figured out how to laser cool and load our atoms continuously rather than staggered in time like almost all other experiments in our field do (i.e., cool and load some atoms, briefly do some science, throw them away, repeat.).”
Before they even got to leverage the very narrow atomic transition during their experiments, the researchers observed that laser light was coming out of the optical cavity and found that it persisted for hours. This fascinating observation was the key inspiration behind their recent work, as they were keen to understand its underlying reasons.
“This was quite a special experiment because normally you try to achieve a specific goal and solve problems along the way,” said Schäfer. “We saw something completely unexpected and initially had no idea what caused it. So, we excluded different possibilities step by step until we finally started to understand what was going on and found out that without us even trying, this lasing mechanism stabilizes the effective frequency of our cavity.”
Ultimately, the researchers realized that the lasing they observed resulted from the absorption of a photon and a subsequent stimulated emission, producing a different momentum state. In other words, they found that 88Sr atoms caught a photon, causing it to recoil and then throw a photon into the cavity, producing the continuous lasing they observed.
“This appears to be the gain mechanism provided by nature when we put energy into the system via our laser-cooling beams,” said Niu.
“However, this gain mechanism also causes atom-heating, which then causes a funny feedback loop that keeps the effective optical cavity frequency to a fixed value, even when we tried our darndest to change it, ” added Thompson.
The recent study carried out by this research group offers new insight into light-matter interactions, which could inform the future development of superradiant lasers. Notably, much of the physics they observed only occurs in continuous, as opposed to cyclic experiments.
“The most interesting lasing regime only appears when starting in a noisier state and then slowly changing the cavity parameters to a less stable regime that is only upheld by the continuous lasing,” explained Schäfer. “So, building a continuously operating cold atom experiment allowed us to see some new effects.”
Inspired by recent work in the field, including this recent study, many researchers interested in atomic and laser physics are now shifting their focus from cyclical to continuous experiments. The resulting continuous operation platforms could pave the way for the introduction of new highly performing technologies, including quantum computing systems and ultranarrow linewidth lasers.
“In the future, we plan to really use the narrow linewidth transition in strontium to build incredibly single-color lasers to explore the world,” added Thompson. “Along the way, we are already seeing lots of interesting things like protecting quantum sensors called matter waves and optical clocks against noise using collective effects or using these same systems to simulate BCS superconductors. We are definitely going to keep very busy!”
More information:
Vera M. Schäfer et al, Continuous recoil-driven lasing and cavity frequency pinning with laser-cooled atoms, Nature Physics (2025). DOI: 10.1038/s41567-025-02854-4.
© 2025 Science X Network
Citation:
Hours-long continuous lasing achieved using laser-cooled strontium atoms (2025, May 5)
retrieved 5 May 2025
from
This document is subject to copyright. Apart from any fair dealing for the purpose of private study or research, no
part may be reproduced without the written permission. The content is provided for information purposes only.